
I think its time to finally round off the long running "Dark Side" trilogy, and like all final chapters it has to be bigger better and more exciting. So sorry but this one is pretty long.
When we left off we had been investigating the properties of the mysterious Dark Matter that permeates the Universe, despite being strange (only interacting through the force of gravity) DM is nevertheless a physical entity, in most theories some form of elementary particle. The next constituent of the Dark Universe is much weirder, being some strange form of energy which exerts a negative pressure on the Universe causing it to expand. Now I think many Astronomers are happy to admit that they are not happy about Dark Energy, everyone seems reasonably happy to admit the existence of Dark Matter, it simply turns up too often on too many scales, but the properties of Dark Energy at present are known from only one or two methods and even then not too accurately, making naturally cautious Astronomers worry about conclusions being drawn from them. Nevertheless the history and implications of a Dark Energy dominated Universe are interesting, so lets have a look at them.
Hubble's observation that essentially all galaxies are receding from us with a velocity that is proportional to the distance between the Milky Way and them was a vital discovery that provided the first evidence that the Universe was expanding. Naturally the idea of a finite age for the Universe (revolutionary at the time) intrigued people, what had happened in the past and what would happen in the future? For a long period of time the belief in the astronomical community was that the Universe started in a Big Bang and that over time the force of gravity would begin to counteract the expansion and slow it.
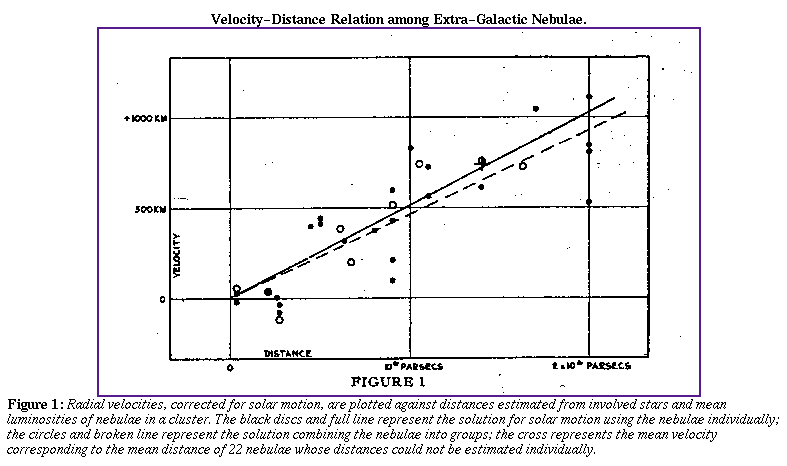
The mathematical formalism that determines the behaviour of the Universal Expansion shows that in this picture there are 3 possibilities for the fate of the Universe:
1. The Universe is not dense enough to halt the expansion and the Universe expands forever.
2. The Universe is exactly dense enough to overcome the expansion when the Universe reaches infinite size. It has the so called "critical density".
3. The Universe is more than dense enough to counteract the expansion and the Universe re-collapses.
Astronomers were therefore keen to determine which of these fates awaited the Universe. To do that they could make use of one of the best standard candles: Type 1a supernovae. In Type 1a supernovae a dense white dwarf that has been accreting matter from a companion suddenly passes over a limiting mass: the Chandrasekhar mass. At this mass the star becomes unstable, undergoes rapid runaway fusion and blows itself apart. Because the Chandrasekhar limit is so precisely defined it means that all Type 1a supernovae have almost exactly the same intrinsic brightness, they also have unique signatures in there spectra that mean they can be separated from other non-uniform SN, hence they can be used as standard candles. Put simply, one supernovae that is observed to be a quarter as bright as another must be twice as far away. To make this technique even more useful these things are bright, as in bright enough to be seen across billions of light years.
In the mid 1990's two groups were using these Type 1a supernovae as standard candles, in attempt to measure how much the expansion of the Universe had slowed since the Big Bang. To do this they combined the physical distance information from the supernovae with the redshift of the galaxy in which the supernovae occurred, this redshift through Hubble's law is also a distance but one that depends on the expansion of the Universe. Hence by plotting one against the other you get a plot of how the expansion of the Universe has changed over time, in essence you are looking for how Hubble's linear relation changes or curves over larger times/distances. When both groups plotted their results they both found the same puzzling result: instead of the rate of the expansion slowing over time it has actually been increasing. Damn it, I'm sure both teams thought as they contemplated all the extra work involved. But very rapidly it probably occurred to them that there's a Nobel prize in it for someone, hence a healthy dose of rivalry between the two teams.

So what could be this strange Dark Energy? To date there are two main contenders, A cosmological constant and quintessence.
The cosmological constant can be thought of as a pressure of a vacuum, particle physics in fact predicts that empty space should have vacuum fluctuations that provide exactly the type of negative pressure required, unfortunately the predictions from particle physics for the level of this pressure are out by up to 120 orders of magnitude, often called the most incorrect prediction in history, oops. The problem is how to cancel out most of this pressure but not all of it, to date no one is sure how to do this. The implications of a cosmological constant are that the expansion will necessarily increase without end, as space is what is causing the expansion the more space there is the more expansion there is. Eventually all structures not gravitationally bound will be separated by so much space light will never be able to pass between them, turning the Universe Dark. In other words in a Milky Way in the distant future, all of the galaxies beyond out local group will slip beyond this distance and disappear forever. Not a very cheerful thought, but hey, who said existence had to be cheerful?
Quintessence is thought to be some sort of particle-like excitation with a possibly dynamical nature. In other words it need not be a constant value per area of space as the cosmological constant, it could vary in strength over time and possibly have different strengths in different areas of the Universe. This is similar to the behaviour of the field that is thought to have caused the intense period of expansion in the early Universe known as inflation. Quintessence could even reverse and cause a contraction of the Universe at some point.
So where does that leave us? What is the eventual fate of the Universe? The truth is that we really don't know for sure, our theoretical knowledge of what is causing the accelerating expansion is not good enough to allow us to determine with certainty what the Universe in the very distant future will be like. The best we can do at present is to try to determine if either of the two cases above can be ruled out. The trick to determining which of these two cases is correct (if either is) is to extend the observations of Type 1a SN to higher redshift and track how the speed of expansion changes. There are many studies ongoing to try to do this to higher and higher precision, expect more interesting results in the coming years.
No comments:
Post a Comment